Borehole Acoustic Televiewer (ATV)
Basic Concept
Borehole-acoustic televiewer (ATV) logging provides continuous, high-resolution, and magnetically oriented ultrasound images of the borehole wall. ATV tools direct ultrasonic signals through fluid-filled boreholes and measure the travel times and amplitudes of the waves that reflect off the borehole wall. The reflected-signal amplitude is proportional to acoustic reflectivity, which is a function of acoustic impedance (i.e., product of density and seismic velocity). An ATV-amplitude log represents variations of borehole-wall acoustic reflectivity, and travel times indicate borehole diameter.
Amplitude and travel-time data can be processed and interpreted together to identify irregularities and structural features that intersect the borehole wall (Williams and Johnson, 2004). ATV images are split (typically on north), unrolled, flattened, and inspected for planar features, which appear sinusoidal. The mid-point depth and orientation (i.e., strike and dip) of the planar features (e.g., fractures, joints, lithologic contacts, and bedding) that intersect the borehole can be determined in processing.
The detection and characterization of fractures is important for fracture-network and geologic characterization, contaminant investigations, and water-resource evaluations. The location of fractures within a borehole can be used to plan flowmeter logging, hydraulic tests, and sampling strategies by directing the placement of tools, packers, and/or pumps. In addition, the results can be used to help interpret other borehole logs and/or surface geophysical surveys.
Theory
Acoustic televiewer logging makes use of the propagation and reflection of ultrasonic-acoustic energy. Early ATV tools employed rotating transducers and collected data that were converted to photographs to be printed, stitched together, and interpreted manually (Zemanek, 1970; Paillet and others, 1990). Advances in tool design have improved the quality, resolution, speed of logging and allow for the collection and storage of digital data (Deltombe and Schepers, 2004).
Modern ATV tools use ultrasonic pulse-echo transducers to emit acoustic signals that travel through the borehole fluid, reflect off the borehole wall, and are measured by the tool. To ensure the signals are reflected, acoustic signals are transmitted normal to (i.e., directly toward) the borehole wall. This is done with either a rotating transducer or, if the tool is equipped with a fixed transducer, a rotating mirror (Williams and Johnson, 2004).
ATV tools emit high-frequency signals (i.e., >1 mega-hertz) to maximize the energy that reflects off the borehole wall and minimize penetration into the formation. In general, hard rocks produce higher-amplitude reflections, and soft rocks produce reflections with little to no amplitude. Waves that encounter enlarged, fractured zones in the boreholes are not reflected and result in low amplitudes and long travel times. Additionally, some signal energy scatters off rough borehole surfaces regardless of material type.
Some advanced ATV tools provide full-scan modes that allow the signal to pass through plastic casing and reflect off the surrounding borehole wall (Deltombe and Schepers, 2004). Mapping fractures with this multi-echo feature is only possible under ideal conditions (i.e., fluid-filled borehole, absence of behind-casing sand pack, and narrow annular space).
ATV images show the measurement depth along the y-axis and azimuth (e.g., 0-360° view) of travel time or amplitude on the x-axis (see figure 1). Typically, ATV logs are collected with 0.01- to 0.02-foot vertical increments and 180-, 270-, 360-, or 720-azimuthal increments. As such, ATV images show borehole-wall acoustic reflectivity and signal reflection-travel time with high vertical and azimuthal resolution, which allows for detailed analysis of structural features.
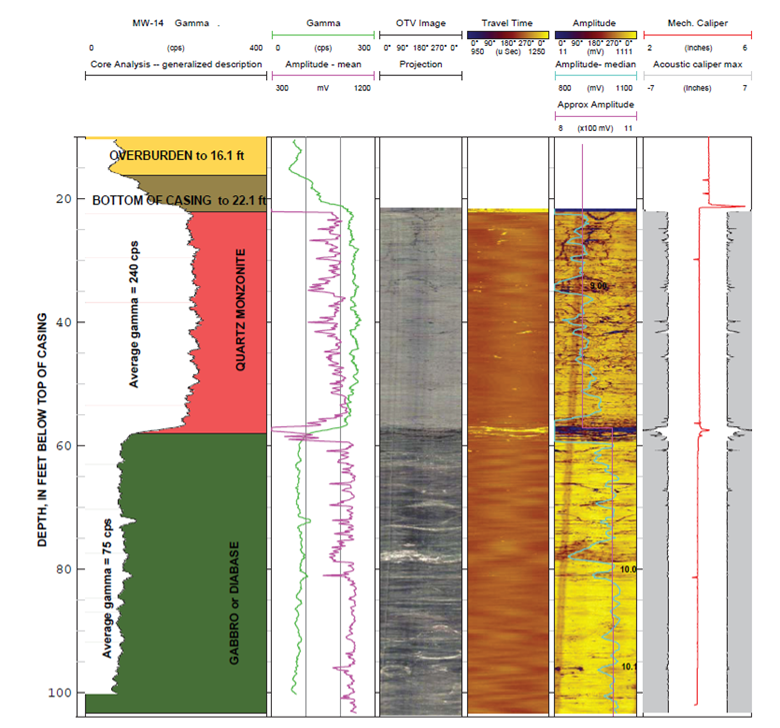
Figure 1. Selected borehole geophysical logs and rock types in borehole MW-14. ATV data shown in logs 1) “Amplitude-mean”, 2) “Travel Time”, 3) “Amplitude”, 4) “Amplitude-median”, 5) “Approx. Amplitude”, and 6) “Acoustic caliper max”. (Johnson and others, 2011)
Applications
Borehole-intersecting planar features (e.g., fractures, bedding, contacts) appear as sinusoids on magnetically ATV oriented images, and their depths and orientations can be calculated and mapped. Strike is normal to the azimuth of the lowest point on the sinusoid (i.e., dip azimuth) and expressed with respect to magnetic or true north. Additionally, apparent dip can be computed as the sine of the amplitude normalized by the borehole diameter.
Typically, borehole deviation logs, which measure the azimuth and inclination of the borehole with depth are collected concurrently with ATV data. The orientation of the borehole deviation and magnetic declination can be applied to the apparent orientations of interpreted features to obtain corrected feature orientations. With such, true dip can be estimated from apparent dip.
The reflection travel-times can be used along with a fluid-velocity estimate to compute an acoustic caliper log that can identify borehole-diameter enlargements and constrictions (figure 1). Additionally, and also shown in Figure 1, the mean- or median-reflectivity values can be computed and related to change in rock type (Johnson and others, 2011). Furthermore, advanced software programs can generate virtual cores whereby optical televiewer logs are wrapped and superimposed on the azimuthally oriented acoustic-caliper log.
The processing and interpretation of digital ATV- images can be done manually with post-processing software or automated structural algorithms (e.g. Wand and others, 2016). Advanced-imaging software packages permit the corrections for magnetic declination, borehole deviation, borehole diameter, and tool decentralization, and poor-quality images can be enhanced by filtering or normalization. Additionally, structural interpretations can be displayed as sine-wave image overlays, tadpole plots, rose diagrams, and stereographic projections.
A successful ATV log requires a fluid-filled borehole so that the ultrasonic waves couple to the borehole wall. Generally, the fluid conductivity and clarity does not affect the signal transmission. However, thick drilling muds may adversely affect the results, so ATV methods are less effective in wells with mudcake on the borehole walls. Additionally, the presence of magnetic minerals and steel casing can “disorient” borehole images, but this is possible to resolve in post processing.
ATV logging is most commonly applied in open-hole fractured rock for water-resource, contamination, and/or geologic-mapping studies (Zoback and Anderson, 1982; Paillet and others, 1990; Williams and Johnson, 2004). Analysis of fracture orientations, break-out zones, and pop-out structures can be used to estimate in situ stress fields (Kingdon and others, 2016; Nei and others, 2013; Zewer and Yassir, 1994; Zoback and others, 1985). Additionally, ATV has been used to estimate porosity, assess casing, and estimate mechanical properties (Chelini, 1998; Rahiman, 2018; Kao and others, 2020). A comprehensive list of ATV applications includes the following:
- Geologic structure mapping (e.g., fractures, bedding, lithologic contacts, foliation, etc.)
- Geotechnical applications (e.g., rock-quality designation, estimating rock properties)
- Supplementing, identifying lost, and orienting core
- Characterizing thin bedding, foliation, joints, and fractures
- Mapping fracture depth and orientation
- Mapping rock units and contacts
- Inspecting casing
- Determining stress field properties and orientations
Examples/Case studies
Chelini, V., Meazza, O., and Verhoeff, E.K., 1998, Relations Between Ultrasonic Amplitude and Petrophysical Characteristics, in Proceedings, European Petroleum Conference: The Hague, Netherlands, Society of Petroleum Engineers, p. 307, doi:10.2118/50606-MS.
Abstract: Empirical evidence suggested that Ultrasonic Amplitude by CBIL tool could be related to formation porosity. A core plug container was designed to investigate this relationship under a variety of controlled conditions. The container holds a number of plugs, radially distributed around the tool transducer. With this experimental setup three different experiments have been carried out. Sandstone, limestone and dolostone samples saturated in water and light hydrocarbon have been used to evaluate the amplitude-porosity relation. The third experimental setup to study the effect of different mud systems on the aforementioned relation has been carried out. The Ultrasonic Amplitude has a distinct linear relation with the core plug porosity for each lithology. The presence of hydrocarbon in pore space increases the CBIL amplitude. The effect of the mud attenuation on the amplitude is independent of the porosity. Measurements of attenuation provide a possible calibration of the amplitude for the downhole environment to the amplitude measured in water. The observed relationships are supported by current theory and model studies. The relation between the CBIL reflection coefficient and plug permeability has also been investigated. The results are applied at downhole acquisitions to evaluate the porosity of the formation using the CBIL amplitude.
Deltombe, J-L., and Schepers, R., 2004, New developments in real-time processing of full waveform acoustic televiewer data: Journal of Applied Geophysics, v. 55, no. 1–2, p. 161-172, doi:10.1016/j.jappgeo.2003.06.013.
Abstract: The new full wave Acoustic Borehole Imager tool (ABI) is a multi-echo (amplitude and traveltime) system that gives optimum performance under a wide range of borehole conditions and is an improvement over existing single echo acoustic televiewer tools. The principle of the multi-echo system is the digital recording of each reflected acoustic wave train. Then, real-time processing of the acoustic data is made by a downhole Digital Signal Processor (DSP) to extract all valuable information, which is further compressed before transmission to the surface such that no special requirements are imposed on data transmission rates or on logging speed. Also, recording of the full acoustic waveform enables major improvements to the dynamic range of the system. Information about echoes from the tool's acoustic window provides the possibility to predict tool generated coherent noise and allows detection of echoes from the borehole wall, which are much smaller than coherent noise signals. When used in a PVC cased borehole, the system can be automatically adapted to record both the reflection at the casing and at the borehole wall. When used inside steel casing, the tool can detect echoes from the inner wall and the outer wall of the steel casing and the resulting data can used to calculate amount inner corrosion, outer corrosion, and remaining casing thickness. If the borehole casing was grouted with cement, information can be gathered about the presence and absence of cement and the quality of cement bonding.
Kao, H-C., Chou, P-Y., and Lo, H-C., 2020, An innovative application of borehole acoustic image and amplitude logs for geotechnical site investigation: Acta Geophysica, v. 68, p. 1821–1832, doi:10.1007/s11600-020-00493-2.
Abstract: Down-hole acoustic televiewer (ATV logging) is a geophysical well-logging device known to be able to reveal underground in-depth image. However, reviewing the current developments in the area of geotechnical engineering, ATV logging data were predominantly used for the purpose of visualizing and calculating the specific structural features in an open borehole, and nothing more. Noticeably, to the majority of engineering applications, it appears that ATV logging played a minor role compared to other geophysical borehole logging tools. Our study does not take it for granted. A systematic study is presented of the significance and consequent applications of ATV logging. The significance of correlation between acoustic amplitude and different geotechnical parameters is discussed and found to be consistent with previous findings. The result tells that ATV logging is actually able to provide timely and ground-truth information during site characterization, if properly used. Additionally, in our study, a simple rock mass evaluation index, Aco-RQD, is proposed, which is applicable to all aspects of geotechnical engineering as long as the continuous ATV logging data are available.
Kingdon, A., Fellgett, M.W., Williams, J.D.O., 2016, Use of borehole imaging to improve understanding of the in-situ stress orientation of Central and Northern England and its implications for unconventional hydrocarbon resources: Marine and Petroleum Geology, v.73, p. 1-20, doi:10.1016/j.marpetgeo.2016.02.012.
Abstract: New interest in the potential for shale gas in the United Kingdom (UK) has led to renewed exploration for hydrocarbons in the Carboniferous age Bowland–Hodder shales under Central and Northern England. Following an incidence of induced seismicity from hydraulic fracturing during 2010 at Preese Hall, Lancashire, the publicly available databases quantifying the in-situ stress orientation of the United Kingdom have shown to be inadequate for safe planning and regulation of hydraulic fracturing. This paper therefore reappraises the in-situ stress orientation for central and northern England based wholly on new interpretations of high-resolution borehole imaging for stress indicators including borehole breakouts and drilling-induced tensile fractures. These analyses confirm the expected north northwest – south southeast orientation of maximum horizontal in-situ stress identified from previous studies (e.g. Evans and Brereton, 1990). The dual-caliper data generated by Evans and Brereton (1990) yields a mean SHmax orientation of 149.87° with a circular standard deviation of 66.9°. However the use of borehole imaging without incorporation of results from older dual-caliper logging tools very significantly decreases the associated uncertainty with a mean SHmax orientation of 150.9° with a circular standard deviation of 13.1°. The use of high-resolution borehole imaging is thus shown to produce a more reliable assessment of in-situ stress orientation. The authors therefore recommend that the higher resolution of such imaging tools should therefore be treated as a de-facto standard for assessment of in-situ stress orientation prior to rock testing. Use of borehole imaging should be formally instituted into best practice or future regulations for assessment of in-situ stress orientation prior to any hydraulic fracturing operations in the UK.
Nie, X., Zou, C., Pan, L., Huang, Z., Liu, D., 2013, Fracture analysis and determination of in-situ stress direction from resistivity and acoustic image logs and core data in the Wenchuan Earthquake Fault Scientific Drilling Borehole-2 (50–1370m): Tectonophysics, v. 593, p. 161-171, doi:10.1016/j.tecto.2013.03.005.
Abstract: After the Wenchuan Earthquake on May 12th, 2008, the Wenchuan Earthquake Fault Scientific Drilling Project (WFSD) was initiated in order to investigate the structure of the fault zones and the mechanism of the earthquake. The WFSD contains four boreholes (WFSD-1, WFSD-2, WFSD-3 and WFSD-4) lying at the maximum displacement locations along the Yingxiu-Beichuan fault zone and the Guanxian-Anxian fault zone, and WFSD-2 is the second borehole and is still being drilled. Core samples, resistivity and acoustic image logging data were acquired from 50 to 1370m. The natural fractures, borehole breakouts, drilling-induced fractures and drilling-enhanced natural fractures were identified from the cores and the image logs and were statistically analyzed. The strikes of the natural fractures systematically vary and can be sorted into four groups according to depth: (1) above 637m, mainly striking ENE–WSW; (2) in the interval of 637–932.6m, striking NNE–SSW; (3) in the interval of 932.6–1200m, directed ENE–WSW then to WNW–ESE, while striking NE–SW from 1030m to 1150m; (4) from 1200m to 1370m, maintaining a strike of WNW–ESE. The natural fractures from 50m to 637m seem to be reverse faults which strike approximately parallelly to the main fault. Two sets of conjugate fractures around 1002.4m indicating subvertical maximum principal paleo-stress direction may be a subordinate structure of the main fault caused by a local stress field, and it reveals the complex stress field of Yingxiu-Beichuan fault zone when the fractures formed. A total of 12 BOs, 2 sets of DIFs and one set of DEFs with an overall length of 30.4m were interpreted from 960m to 1370m in WFSD-2. The average SHmax orientation interpreted for WFSD-2 (960–1370m) is 120.7°–300.7°N (i.e. WNW–ESE) with the standard deviation of 9.2° and it is consistent with the stress status of Yingxiu-Beichuan fault zone which is one of the main fault zones in the 2008 Wenchuan Earthquake.
Rahiman, T.I.H., 2018, Digital imaging of geotechnical boreholes: benefits and risks: Australian Geomechanics Journal, v. 53, no. 3, p. 153-161.
Abstract: In recent years, the use of borehole televiewers has become common in geotechnical site investigations in Australia, particularly those involving engineering structures in rock. Borehole televiewers can rapidly and efficiently generate vast lengths of high resolution digital imagery of borehole walls and borehole survey data. These extraordinary geo-referenced images of the subsurface present several major advantages for ascertaining in situ geotechnical information over conventional geotechnical core logging. The mystery of core loss zones can be resolved. Rock fabric directions do not require relatively unreliable oriented core measurements. Deviated boreholes can now be correctly placed in 3D ground models. Borehole principal stress directions can be assessed and the painstaking task of logging rock defects at mm scale can be done patiently onscreen. As with any ground test procedure, there are potential bad practices, hidden sources of error and some drawbacks in the results of televiewer data. Poor results can be due to the wrong choice of tools, overlooking of tool calibration checks, and lack of care with tool centralisation. Major sources of inaccuracies can arise from neglecting corrections of borehole diameter and trajectory variations. Quality issues and erroneous readings of borehole geometry aside, the televiewer images present factual and unbiased depictions of the boreholes. Yet analysis of the images to extract information of geotechnical interest can be subjective, requires input from an engineering geologist or geotechnical engineer and verification with rock core data. © 2018 Australian Geomechanics Society.
Srinivas, K.N.S.S.S., Rao, M.S., Kishore, P.P., Gopinadh, D., Raza, H., Arora, K., and Satyanarayana, H.V.S., 2018, Delineation of Fractures through Acoustic Televiewer Log: Journal of the Geological Society of India, v. 91, p. 569–574, doi:10.1007/s12594-018-0906-x.
Abstract: Boreholes are the only sources for direct measurements of geological and geophysical characteristics of the shallow subsurface of the earth. Borehole imaging tool “Acoustic Televiewer (ATV)” is an advanced probe, which records 3D image of the bore hole wall and is used to obtain oriented images of bore hole and provides substantial information regarding lithology, structural information, detection of fractures and casing of the borehole. The images are highly sensitive to the presence of fractures, the delineation of which becomes accurate and reliable based on these acoustic images. Features identified on log-derived images can be correlated with core samples or can be used as substitute in the poor core recovery zones. In the present study, ATV log from a deep borehole drilled at Khadi Kolavan in Koyna-Warna region of Ratnagiri district, Maharashtra located west of the Western Ghat escarpment in the Deccan Volcanic province, India, is used for interpretation of structural characteristics of the formation. The same was correlated with other conventional logging methods such as resistivity and full waveform sonic (FWS) as well as core logging and rock quality designations (RQD) which generated new understanding of the basement and basalt cover in this region.
Wang, C., Zou, X., Han, Z., Wang, Y., and Wang, J., 2016, An automatic recognition and parameter extraction method for structural planes in borehole image: Journal of Applied Geophysics, v. 135, p. 135-143, doi:10.1016/j.jappgeo.2016.10.005.
Abstract: As a breakthrough in borehole imaging technology, digital panoramic borehole camera technology has been widely employed. The high-resolution panoramic borehole images can accurately reproduce the geometric features of structural planes. However, the detection of these features is usually done manually, which is both time-consuming and introduces human errors. To solve this problem, this paper presents a method for the automatic recognition and parameter extraction of borehole geometric features of camera images. In this method, the image's gray and gradient level, and also their projection on the depth axis are used to identify the locations of structural planes. Afterwards, iterative matching is employed by using a template of sinusoidal function to search for structural planes in the identified image blocks. Finally, optimal sine curves are selected as the feature curves of structural planes, and their related parameters are converted into structural plane parameters required for engineering, such as their positions, dip directions, dip angles and fracture widths. The method can automatically identify all of structural planes throughout the whole borehole camera image in a
continuous and rapid manner, and obtain the corresponding structural parameters. It has proven highly reliable, accurate and efficient.
Williams, J.H., and Johnson, C.D., 2004, Acoustic and optical borehole-wall imaging for fractured-rock aquifer studies: Journal of Applied Geophysics, v. 55, no. 1-2, p. 151-159, doi:10.1016/j.jappgeo.2003.06.009.
Abstract: Imaging with acoustic and optical televiewers results in continuous and oriented 360j views of the borehole wall from which the character, relation, and orientation of lithologic and structural planar features can be defined for studies of fractured-rock aquifers. Fractures are more clearly defined under a wider range of conditions on acoustic images than on optical images including dark-colored rocks, cloudy borehole water, and coated borehole walls. However, optical images allow for the direct viewing of the character of and relation between lithology, fractures, foliation, and bedding. The most powerful approach is the combined application of acoustic and optical imaging with integrated interpretation. Imaging of the borehole wall provides information useful for the collection and interpretation of flowmeter and other geophysical logs, core samples, and hydraulic and water-quality data from packer testing and monitoring.
References
Chelini, V., Meazza, O., and Verhoeff, E.K., 1998, Relations Between Ultrasonic Amplitude and Petrophysical Characteristics, in Proceedings, European Petroleum Conference: The Hague, Netherlands, Society of Petroleum Engineers, p. 307, doi:10.2118/50606-MS.
Deltombe, J-L., and Schepers, R., 2004, New developments in real-time processing of full waveform acoustic televiewer data: Journal of Applied Geophysics, v. 55, no. 1–2, p. 161-172, doi:10.1016/j.jappgeo.2003.06.013.
Johnson, C.D., Mondazzi, R.A., and Joesten, P.K., 2011, Borehole geophysical investigation of a formerly used defense site, Machiasport, Maine, 2003–2006: U.S. Geological Survey Scientific Investigations Report 2009–5120, 333 p., doi:10.3133/sir20095120.
Kao, H-C., Chou, P-Y., and Lo, H-C., 2020, An innovative application of borehole acoustic image and amplitude logs for geotechnical site investigation: Acta Geophysica, v. 68, p. 1821–1832, doi:10.1007/s11600-020-00493-2.
Kingdon, A., Fellgett, M.W., Williams, J.D.O., 2016, Use of borehole imaging to improve understanding of the in-situ stress orientation of Central and Northern England and its implications for unconventional hydrocarbon resources: Marine and Petroleum Geology, v.73, p. 1-20, doi:10.1016/j.marpetgeo.2016.02.012.
Nie, X., Zou, C., Pan, L., Huang, Z., Liu, D., 2013, Fracture analysis and determination of in-situ stress direction from resistivity and acoustic image logs and core data in the Wenchuan Earthquake Fault Scientific Drilling Borehole-2 (50–1370m): Tectonophysics, v. 593, p. 161-171, doi:10.1016/j.tecto.2013.03.005.
Paillet, F.L., Barton, C., Luthi, D., Rambow, F., and Zemanek, J., 1990, Borehole imaging and its application in well logging – A review, in Paillet, F.L., and others, eds., Borehole Imaging: Society of Professional Well Log Analysts, p.1-23.
Rahiman, T.I.H., 2018, Digital imaging of geotechnical boreholes: benefits and risks: Australian Geomechanics Journal, v. 53, no. 3, p. 153-161.
Srinivas, K.N.S.S.S., Rao, M.S., Kishore, P.P., Gopinadh, D., Raza, H., Arora, K., and Satyanarayana, H.V.S., 2018, Delineation of Fractures through Acoustic Televiewer Log: Journal of the Geological Society of India, v. 91, p. 569–574, doi:10.1007/s12594-018-0906-x.
Wang, C., Zou, X., Han, Z., Wang, Y., and Wang, J., 2016, An automatic recognition and parameter extraction method for structural planes in borehole image: Journal of Applied Geophysics, v. 135, p. 135-143, doi:10.1016/j.jappgeo.2016.10.005.
Williams, J.H., and Johnson, C.D., 2004, Acoustic and optical borehole-wall imaging for fractured-rock aquifer studies: Journal of Applied Geophysics, v. 55, no. 1-2, p. 151-159, doi:10.1016/j.jappgeo.2003.06.009.
Zemanek, J., Glenn, E.E., Norton, L.J., and Caldwell, R.L., 1970, Formation evaluation by inspection with the borehole televiewer: Geophysics, v. 35, no. 2, p. 254-269, doi: 10.1190/1.1440089.
Zewer, M.D., and Yassir, N.A., 1994, Borehole breakout interpretation in the Gulf Coast, offshore Louisiana, in, Nelson, P.P., and Laubach, S.S., eds., Rock Mechanics Models and measurements challenges from industry: Rotterdam, The Netherlands, A.A. Balkema, p. 225-232.
Zoback, M.D., and Anderson, R.N., 1982, Ultrasonic borehole televiewer investigation of oceanic crustal layer 2a, Costa Rica rift: Nature, v. 295, p. 375-379, doi: 10.1038/295375a0.
Zoback, M.D., Moos, D., Mastin, L., and Anderson, R.N., 1985, Well bore breakout and in situ stress: , Journal of Geophysical Research: Solid Earth, v. 90, no. B7, p. 5523-5530, doi: 10.1029/JB090iB07p05523.